An international consortium of physicists headed by Thibaut Lacroix, Brendon W. Lovett, and Alex W. Chin from institutions in Germany, UK, and France has brought forth an illuminating research on Quantum Nanodevices. The research digs into the hitches faced in the real-world application of quantum technologies (QT) and how those can be turned around to exploit the precision and ultra-efficient mechanisms seen in biological nanomachines, such as enzymes and photosynthetic proteins.
Nanodevices that exploit quantum effects play a highly significant role in future quantum technologies. However, their performance is impeded by “decoherence” – a concept in quantum mechanics where quantum states lose their quantum nature due to environmental interactions. This problem is compounded as devices become multi-faceted: when they contain multiple units, the respective local environments begin to overlap, causing environmentally mediated decoherence phenomena on new time-and-length scales.
These complex and inherently non-Markovian dynamics could present a challenge in scaling QT. However, the environmental ability to transfer signals and energy could enable sophisticated spatiotemporal coordination of inter-component processes. This suggests the potential to harness this ability, as seen in biological nanoscale marvels such as enzymes and photosynthetic proteins.
Using numerically exact many-body methods – specifically tensor networks – the researchers produced a comprehensive quantum model. This model allowed them to examine how propagating environmental dynamics could instigate and direct evolution in spatially remote, non-interacting quantum systems. They also demonstrated how energy dissipated into the environment could be remotely harvested to create transient excited or reactive states.
Interestingly, they identified how reorganization triggered by system excitation could qualitatively and reversibly alter the downstream kinetics of a functional quantum system. The researchers used complete system-environment wave functions to gain insights into the microscopic processes underlying these phenomena, offering new perspectives on how they could be leveraged for energy-efficient quantum devices.
Despite the limiting factors of decoherence, this groundbreaking study reveals that as quantum systems interact with a common environment, it opens up new opportunities for control, sensing, and cross-talk mechanisms. This understanding can have profound implications in designing future quantum technologies and devices, thus paving the way to engineering more efficient, resilient QTs.
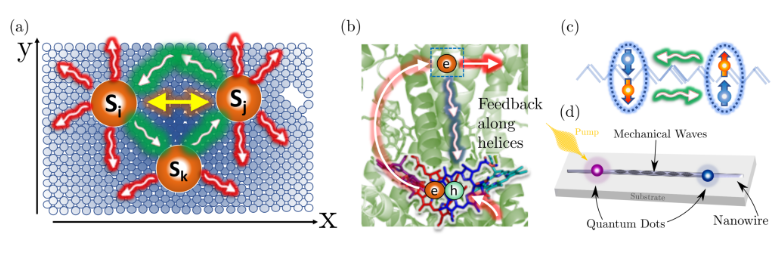
Examples in 1D:
(b) in photosynthetic reaction centers, pigments are held by a protein scaffold that can dissipative mediate vibrations and structural reorganization to coordinate exciton (electron-hole pair) splitting, electron transfer and hole refilling in different locations (separated by 4 − 5 nm) on timescales from the fs to the μs.
(c) Spin-entangled triplet-pairs generated by intramolecular singlet fission interact strongly through the vibrational wave packets of the molecular backbone structure, as in polydiacetylene.
(d) A pair of uncoupled quantum dots (QDs) are encapsulated in a nanowire. Exciting one of them thus excites the mechanical modes of the wire. These distortions propagate and could interact with the other QD.
Information Box:
– Non-Markovian dissipation refers to the process of energy transfer among quantum systems influenced by their environment’s memory effects.
– Decoherence is a major obstacle faced in practical quantum computing, where environmental ‘noise’ creates disturbances in the delicate quantum states.
– Quantum nanodevices are expected to revolutionize industries like computing, communication, and sensing by exploiting the exceptional features of quantum mechanics.
– The study uses a full quantum model along with tensor network methods, advanced computational tools used to study quantum systems, to uncover new mechanisms for QTs.
Reference 1: “From Non-Markovian Dissipation to Spatiotemporal Control of Quantum Nanodevices,” Quantum 8, 1305 (2024), Thibaut Lacroix, Brendon W. Lovett, and Alex W. Chin.
Reference 2: Institut für Theoretische Physik und IQST, Universität Ulm, Germany.
Reference 3: School of Physics and Astronomy, University of St Andrews, UK.
https: //quantum-journal.org/papers/q-2024-04-03-1305/